Introduction
Cerebral autoregulation (CA) is the process through which the brain maintains a sufficient cerebral blood flow (CBF) despite varying cerebral perfusion pressure (CPP).1, 2 When systemic BP varies, the cerebral vasculature's inherent CA protection mechanism maintains CBF stability. There are two main areas of CA study. Static CA establishes the arterial blood pressure (ABP) autoregulatory limits under various, somewhat stable conditions (working a few minutes to many hours).1, 2, 3 Dynamic CA, on the other hand, includes spontaneous physiological changes in ABP and CBF (during transient changes in mean arterial pressure (MAP) (for instance, with changes in posture), occurring over seconds or occurring after deflation of a cuff to pool or release blood from one or more extremities quickly).2, 3, 4 According to experiments, brain circulation is more effective at absorbing lower-frequency blood pressure variations (0.20 Hz) than higher-frequency fluctuations (>0.20 Hz).1, 2, 3, 4, 5 The autonomic nerve system (ANS), among other physiological pathways, may impact CA disturbances. Cholinergic and adrenergic nerve fibers innervate the cerebral vascular system.5, 6 Due to a variety of factors, including the complexity of the ANS and cerebrovascular interactions, measurement flaws, differences in methods used to evaluate the ANS concerning CBF, and experimental strategies that can or cannot shed light on the sympathetic control of CBF, the role of the ANS in regulating CBF is hotly debated.6 Both sympathetic and parasympathetic fibers densely innervate the cerebral vasculature, although it is still unclear how they affect CBF by regulating the diameter of the vessels.7, 8 It is particularly uncertain whether sympathetic modulation actively contributes to CA or merely represents an additional, inevitable element that regional vasoconstrictive/vasodilative mechanisms must balance. However, whether any subsidiary variable mediates the observed frequency-dependent influences or vagal control directly influences the cut-off of CA's high pass dynamic feature is unclear. To comprehend the mechanisms underlying the reported crosstalk between the controls of the heart and the cerebrovascular system, define more precisely the frequency bands to compute spectral CA markers, and develop models more in line with physiology to describe the behavior of CA; it may be helpful to study the involvement of the ANS on CA.9 The review investigates how the ANS affects CA, which could have a significant clinical impact on the diagnosis and treatment of pathological diseases. In this review, the authors pointed out the answers to the questions: What is cerebral autoregulation, and what are the parameters to study cerebral hemodynamics? What is reflex control of arterial pressure? What is the autonomic nervous system concerning CBF Regulation? What are the systemic parameters used to quantify autonomic functions? What are the assessment methods of Autonomic Function? What are the Autonomic Challenges and Methods of Evaluation? What is the clinical significance of autonomic insufficiency?
Methods for searching
We explored the literature related to cerebral autoregulation, cerebral hemodynamics, reflex control of arterial pressure, autonomic nervous system, cerebral blood flow, autonomic functions, assessment methods of autonomic function, and autonomic challenges with methods of evaluation. We browsed Cochrane, PubMed, Scopus, Embase, and Google Scholar databases. Keywords used are (cerebral autoregulation) (Blood Pressure[MeSH Terms]) AND (Cerebral autoregulation[MeSH Terms])) AND (brain[MeSH Terms])) OR (cerebral perfusion pressure)) OR (arterial blood pressure[MeSH Terms])) OR (posture[MeSH Terms])) OR (Cholinergic)) OR (adrenergic nerve fibers)) OR (reflex control of arterial pressure)) OR (autonomic functions)) OR (autonomic Nervous System[MeSH Terms]).
Cerebral autoregulation
The mechanisms that keep CBF constant while blood pressure varies are called cerebral autoregulation.1, 2 Although the underlying physiological mechanisms are unknown, cerebral autoregulation is frequently divided into static and dynamic components. The difference between static and dynamic cerebral autoregulation, which operates over many minutes to hours and depicts the steady-state relationship between mean artery pressure (MAP) and CBF, is experimental rather than physiological. Contrarily, dynamic cerebral autoregulation typically refers to the cerebral pressure-flow relationship seen during brief variations in MAP (such as those caused by changes in posture).2, 3, 4, 5, 6, 7 Although these two measurements operate on a continuum, brain circulation appears to be more effective at dampening blood pressure variations of lower frequency (0.20 Hz) than of higher frequency (>0.20 Hz).10
Parameters for cerebral hemodynamics
A few key metrics are frequently used to describe hemodynamics. The distinction between global and localized hemodynamic parameters is a crucial idea, and for instance, there is a huge difference between regional and global CBF (or perfusion). Cerebral blood volume (CBV) refers to the proportion of brain blood vessel volume to brain tissue volume. CBV values are 4 ml of blood per 100 ml of tissue at rest.11, 12, 13, 14, 15 The CBV (aBV) arterial component, equivalent to 30%, contributes approximately 1 ml of cerebral blood vessels per 100 ml of tissue.16 The amount of oxygen utilized, tissue volume, and time are all represented by the cerebral metabolic rate of oxygen (CMRO2). The cerebral oxygen metabolic rate is influenced by oxygen extraction fraction, CBF, and arterial oxygen concentration.17 The brain uses 20% of baseline oxygen at rest and relies on the oxygen-dependent metabolism of glucose.18, 19, 20 Cortical rest values of CMRO2 are about 3.5 ml of O2/ 100 g of brain tissue/ minute.21 Cerebral perfusion (CBF) is the blood volume that perfuses a defined mass of brain tissue/ time (ml/100g/min). Average CBF values in humans are 40-60 ml/100g/min range15, 22 and are about three times lower in white matter than in grey matter (average values: 20 mL/100g/min and 70 mL/100g/min, respectively).
The autonomic nervous system
All visceral functions necessary for the organism to maintain homeostasis are under the direction and regulation of the autonomic nerve system. Neurohormones that are secreted operate at specific receptors in the smooth muscles of the heart, gastrointestinal tract, respiratory tract, eyes, and skin to regulate these processes.11, 12 The autonomic nervous system comprises both the sympathetic and parasympathetic systems. Together with the central autonomic network and its numerous connections in the brain stem, these two divisions comprise the peripheral autonomic nervous system, which functions.12
Sympathetic nervous system
The inter mediolateral cell section of the spinal cord segments from T1 to L3 contains the nerve cells of the sympathetic nervous system. The paravertebral sympathetic trunk, which is made up of synaptic ganglia and runs close to the vertebral bodies, is formed by axons from these cell bodies that follow the ventral rami of the segmental nerves, escape with these nerves via the short myelinated preganglionic fibers, and create the segmental nerves.10, 11 The sympathetic ganglia generate long postganglionic fibers that innervate the target organs. Particular alpha and beta-adrenergic receptors can be found in target end organs. While the postganglionic neurons of the sympathetic nervous system release noradrenaline as the main neurotransmitter at each of their target organs, the preganglionic neurons of the sympathetic nervous system release acetylcholine at nerve endings in their respective ganglia.12
Parasympathetic nervous system
Specific cell columns constitute this branch of the autonomic nervous system in the segments S2–S4 of the sacral spinal cord, as well as the special nuclei of the cranial nerves III, VII, IX, and X. Long preganglionic nerves innervate the synaptic ganglia, which are present within or close to the effector organ.10, 11, 12 Acetylcholine is the primary neurotransmitter in the ganglia and effector organs. There are two types of receptors engaged in neurotransmission: muscarinic and nicotinic. The two divisions of the peripheral autonomic nervous system do not innervate all bodily organs; even when they are, the end-organ only generally responds to one at a time. Table 1 lists the different actions produced due to peripheral autonomic activation.12, 13
Reflex control of arterial pressure
Since arterial pressure variations may be incompatible with life, arterial pressure is one of the physiological factors in the human body that is closely regulated. One of the ANS's key functions is to counteract abrupt variations in arterial pressure. A quick reduction in arterial pressure is characterized by activating the sympathetic branch and suppressing the vagal tone within the ANS.11, 12, 13 As a result of such processes, the total peripheral resistance rises, the veins are constricted to aid venous return to the heart, the heart rate increases, and the heart's contractile force increases. These actions increase arterial pressure. Conversely, the ANS acts oppositely when the arterial pressure rises. Numerous reflex neural control mechanisms constantly come into play to keep the arterial pressure at or close to normal.12, 13 Due to its fast activation, the baroreflex (BR), one of the several reflex reactions, is probably the most important of these regulatory systems. From this point forward, blood pressure oscillations are controlled within the physiologically acceptable ranges by a crucial neuronal negative feedback process integrating baroreceptor and cardiopulmonary responses13 Pressure-sensitive receptors found in big systemic veins, the heart's atria, and the pulmonary vasculature (low-pressure baroreceptors) and arterial baroreceptors (high-pressure baroreceptors) make up afferent pathways. The carotid sinuses, close to the right subclavian artery's origin, and the aortic arch all contain high-pressure baroreceptors.14, 15, 16, 17, 18 The glossopharyngeal nerve (IX cranial nerve) transmits carotid baroreceptor input to the earliest synapses in the brainstem's Nucleus Tractus Solitarii (NTS). Baroreceptor data from the aortic arch is transmitted through the aortic depressor nerve, which connects to the vagus nerve (X cranial nerve) and ascends to the NTS. Cardiopulmonary receptors from the heart and lungs innervate the same NTS nuclei through the X nerve—excitation-inducing projections from the NTS synapse in the nucleus ambiguous, caudal ventrolateral medulla, and the vagus. The primary sympathetic output nucleus, which is situated at the level of the rostral ventrolateral medulla (RVLM), receives inhibitory projections from the caudal ventrolateral medulla (CVLM) concerning muscle sympathetic nerve activity (MSNA).14, 21 This type of innervation causes the sympathetic outflow to the muscle vascular bed to decrease. As a result, at the RVLM, the excitatory signal from the baroreceptor afferents is inverted (Figure 1). Stretching of the vascular wall caused by elevated blood pressure (BP) increases the frequency of baroreceptor discharge, which in turn causes less sympathetic activation and more parasympathetic activity. As a result of this combined response, blood pressure, and heart rate decrease. As the blood pressure decreases, the variables shift in the other direction.15, 16, 17, 18, 19, 20
Autonomic Nervous System Concerning CBF Regulation
The cerebral circulation is filled with sympathetic nerve fibers. While the body is at rest, sympathetic nerve activity may not significantly control CBF.20, 23 However, sympathetic nerve activity can release neurotransmitters like norepinephrine and neuropeptide-Y into the perivascular zone and modify vascular tone (vasoconstriction).
Due to a mechanism known as neurovascular coupling (NVC), CBF might increase in response to brain activation to satisfy the increasing metabolic demand. During synaptic transmission, this connection was mediated by several substances, including CO2, O2, K+, Ca2+, H+, and adenosine.24 The breakdown of adenosine triphosphate results in the production of adenosine, which might lead to pial arterioles dilating due to glutamate.25 Because astrocytes are linked, increased Ca2+ levels caused by electrical neuronal activity or high glutamate levels at their end feet may cause vasodilation.26 Capillaries can enlarge even as a result of pericyte hyperpolarization.26
Systemic parameters used to quantify autonomic functions
Heart rate
The heart rate is commonly stated in beats per minute (bpm), also known as the number of beats per unit of time. Heart rate is frequently utilized as a diagnostic and monitoring criterion. The variation between R-R intervals is called heart rate variability (HRV).
Blood Pressure
The blood pressure alternates between its peak (systolic) and lowest (diastolic) levels with each heartbeat. Systolic pressure is the arterial pressure at its highest point at peak ventricular ejection, while diastolic pressure is the arterial pressure at its lowest point right before ventricular ejection starts during the cardiac cycle.
There are numerous invasive and non-invasive methods for measuring arterial blood pressure. A needle is inserted into an artery during invasive blood pressure tests to measure arterial pressure. Using non-invasive methods has various benefits, such as reducing patient discomfort and requiring little technical expertise to operate non-invasive measurement gear. However, the main drawback of adopting a non-invasive approach is the possibility of less accurate blood pressure readings. The auscultatory approach and the volume clamp technique, which uses a finger cuff and is based on the Penaz principle, were both widely utilized non-invasive blood pressure monitoring techniques in this study.
Heart rate variability
A physiological phenomenon is the heartbeat variation from one beat to the next. It gauges how much the RR interval, also known as the interbeat interval, fluctuates. HRV is evidence of the autonomic control of heart rate. 27 High HRV values are created when autonomic control of heart rate is effective, and this quantity indicates effective parasympathetic activity. Low HRV total power levels indicate sympathetic activation. Figure 2
The interaction of the accelerating and decelerating actions of sympathetic and parasympathetic innervation as well as by the natural rhythmicity of the cardiac pacemaker tissue, determines the interbeat interval, or the interval between two R waves.28 Respiratory sinus arrhythmias are physiological changes in heart rate that happen during breathing. The renin-angiotensin system, hormonal shifts, thermoregulation, and circadian rhythm all impact HRV. Baroreflex processes and variations in blood pressure also influence it. 28, 29
Respiratory sinus arrhythmia modulation via baroreceptor reflexes
Tachycardia on inhalation and bradycardia on exhalation result in respiratory sinus arrhythmia. Increased venous return on inhalation triggers the Bainbridge reflex, which results in tachycardia and, eventually, respiratory sinus arrhythmia. Increased venous return after inhalation causes the heart muscle to lengthen initially. 28, 29 According to the Frank-Starling principle, lengthening the cardiac muscle increases the force of contraction, which raises the stroke volume and engages the baroreflex system, counteracting the tachycardia-inducing effects of the Bainbridge reflex. The balance of the Bainbridge and baroreceptor reflex determines the severity of the respiratory sinus arrhythmia. 29
Frequency domain parameters
Power spectral analysis
The quick Fourier Transform Algorithm examines the power spectrum of heart rate variations. The short-term HRV is studied using these two techniques. The power spectral density analysis, which provides details on the strength of the heart's sinus rhythm and a breakdown of various heart rate amplitudes and frequencies, can be used to identify periodic fluctuations in heart rate. Discrete peak frequencies can be observed thanks to the Fast Fourier Transform (FFT) 30, 31 The results of FFT can be converted in numerous ways into bands with various frequencies. The standardized frequency bands for HRV are described below:
Total Power (TP): It has a spectral range of 0 and 0.4 Hz. It generally represents autonomic activity. The unit for total power is the millisecond square (ms2).
Very low frequency (VLF): The power spectral range of VLF is 0.0033 to 0.04 Hz. The millisecond square (ms2) is the unit of measurement. This component is associated with the activity of the renin-angiotensin mechanism, thermoregulation, hormonal regulation, and local factors.
Low frequency (LF): The power spectral frequency range of LF is 0.04 to 0.15 Hz. LF reflects both the sympathetic and parasympathetic components. Sympathetic activity may be predicted by LF as a whole. The LF is measured in millisecond squares (ms2).
High-frequency power (HF): The high-frequency range is 0.15 to 0.4 and is a parasympathetic activity marker. Because it experiences changes with breathing, it is also known as the respiratory band. Millisecond squared (ms2) is the unit of measurement.30, 31, 32, 33
The LF/HF Ratio (LF/HF): A larger ratio indicates sympathetic dominance, whereas a ratio with lower values indicates parasympathetic dominance. (Figure 3)
Blood pressure variability
BP is characterized by strong short- and long-term oscillations, including changes from hour to hour, minute to minute, and beat to beat, in addition to diurnal changes and changes over weeks to years. 30, 31 Blood pressure variation over time is blood pressure variability (BPV) brought on by interactions between environmental and behavioral factors, cardiovascular control mechanisms like brain centers, neural reflexes, and humoral effects. 32
On a beat-by-beat basis, the heart rate is modulated by the baroreceptor reflex, the vascular, myogenic response, and the creation of nitric oxide by the vascular endothelium 33, 34 Continuous, direct intra-arterial recordings are the most accurate method for assessing these short-term BPVs. Spectral analysis of beat-by-beat BPV is used to evaluate the proportional contribution of the aforementioned neurohormonal signals to BPV. 35 Increased sympathetic drive, diminished arterial baroreflex, alterations in behavior and emotion, and ventilation causes an increase in variations in beat-to-beat blood pressure. The most common and frequently used non-invasive beat-to-beat monitoring devices combine the Penaz finger cuff or volume clamp technique with the Wesseling physiological criteria to provide precise, non-invasive, and continuous blood pressure assessments.36, 37, 38
Power spectral analysis of BPV
The oscillations at very low frequency (VLFO: 0.003- 0.04Hz), low frequency (LFO: 0.04-0.15 Hz), high frequency (HFO: 0.15-0.40 Hz), total power spectral density (PSD; 0.04 Hz), and LF/HF ratio were among the frequency components of BPV. 35, 39, 40 Evidence suggests that LFO BPV measures changes in sympathetic activity, whereas myogenic vascular function, the renin-angiotensin system, and endothelium-derived nitric oxide are shown to impact BPV at VLFO. 35, 40 Moreover, LF/HF ratio is established as a marker of sympathetic vascular activity, and HFO BPV is mostly affected by variations in cardiac output. 39
Baroreflex resonance and Mayer waves
Baroreflex loop means that a change in either function results in a change in the other because the heart rate and blood pressure fluctuate in a closed loop. Heart rate rises due to elevated blood pressure via baroreceptor activation and vice versa.
As the blood pressure starts to vary, the baroreflex cardiac response (HR change) follows in a fraction of a second. The heart rate fluctuates once every ten seconds for one pressure stimulus.41, 42
As a result, oscillations at 0.1 Hz constitute the Mayer waves generally. The delay duration (inertia) ranges between 4 and 6.5 s. Thus the frequency of Mayer waves is between 0.075 and 0.12 Hz (in the LFO-BPV range). 43 These 10-second-periodic blood pressure oscillations are closely associated with synchronous oscillations of efferent sympathetic nerve activity and are amplified in sympathetic activation. 44
Mayer wave-related hemodynamic oscillations have also been seen in the human cerebral circulation. 45
Traube Herring Waves
The mechanical connection between cardiac output and breathing leads to respiratory-induced pressure oscillations. 46 Each inspiration causes an increase in negative intrathoracic pressure, which causes the right ventricle's venous return to increase quickly. This decreases cardiac output and lowers arterial pressure. 47 During expiration, the reverse takes place. Traube-Hering waves are these oscillations that are "out of phase" (180°) with the respiratory flow. These oscillations at spontaneous breathing frequency range mostly between 0.15 and 0.4 Hz and set the upper and lower bounds for BPV HFOs. These oscillations assume and shift to a low-frequency oscillation of BPV during paced breathing at 0.1 Hz. 48
Time domain beat-by-beat parameters BPV
Mean-The average blood pressure (SBP, DBP, or MBP)
SD - Standard deviation (SD) for all intervals of BP (SBP, DBP, or MBP).
RMSSD stands for the square root of mean squared differences (SBP, DBP, or MBP)
The quantification of HRV and BPV revealed that the subject's RRI variability (SD and RMSSD) was higher in supine (horizontal) posture and lower in vertical (standing) posture. The SD and RMSSD of SBP and DBP vary in opposite directions, being larger when the subject was upright and lower when the subject was supine.49
Long-term BPV- Changes in arterial compliance, adjustments to the patient's blood pressure-lowering medication, errors in blood pressure measurements, and seasonal change produce observed variations in blood pressure from one day, week, or month to the next.32
Assessment of Autonomic Function
A variety of tests are available to gauge autonomic performance. These tests can be categorized based on the system involved; the neurotransmitters engaged, the functions exposed, the reactions induced, the sensory modality involved, and the degree of invasiveness. Cardiovascular autonomic function testing assesses autonomic responsiveness, including the Deep Breathing Test, Valsalva Manoeuvre, Isometric Handgrip Test, Cold Pressor Test, and Lying to Standing Test/Head-up Tilt Test. All of these tests for autonomic reactivity are reflex-based, and both the stimulus and the response are observably measured. 50
Assessment of cardiovascular autonomic functions
Test for cardiovagal reactivity (parasympathetic innervation): The heart rate (HR) response to deep breathing, the Valsalva ratio, and the HR reaction to standing
Adrenergic reactivity: heart rate (HR) and blood pressure (BP) responses to tilting up or active standing, prolonged hand gripping, and beat-to-beat blood pressure (BP) responses to the Valsalva Maneuver51, 52, 53, 54, 55
Cardiovagal heart rate tests
Paced breathing at 0.1 Hz
In contrast to spontaneous breathing, which occurs between 12 and 20 cycles per minute in adults and typically has a larger breathing amplitude, breathing is performed at a slower rate (around six cycles per minute, or CPM). 51, 52 The efferent and afferent systems are formed via vagal pathways. 51, 53 The natural response is for the heart rate to rise with inspiration and fall with expiration. The term sinus arrhythmia defines this shift in heart rate with breathing. 54, 55 The variation in heart rate with respiration is mediated in a significant way by the vagal innervation of the heart. Pulmonary stretch receptors, cardiac mechanoreceptors, and baroreceptors regulate changes in heart rate.
Valsalva maneuver
The Valsalva Maneuver forces air via an open glottis into a mouthpiece connected to a manometer. Pressure in the manometer should be maintained at 40 mmHg for 15 seconds. As Hamilton, Woodbury, and Harper proposed, responses to the Maneuver can be divided into four phases. 56 Phase I and III blood pressure variations are entirely due to mechanical causes. Phase II heart rate increases are first caused by vagal withdrawal and then by an increase in sympathetic outflow. Baroreflex mediates the reduction in heart rate in response to Phase IV overshoot (vagal). To evaluate the responses Valsalva ratio is calculated by dividing the highest HR produced by the Valsalva Maneuver by the lowest HR experienced after the move. A value of more than 1.2 is considered as normal. 51
Lying to standing test
On standing, the HR responses show an initial tachycardia at 3 seconds and then at 12 seconds, followed by bradycardia at 30 seconds. The initial cardio acceleration is an exercise reflex. After that, the blood pressure drops due to the venous decline brought on by blood pooling in the lower limbs by the shift in posture from reclining to standing.50 The baroreflex is triggered by a drop in blood pressure, which causes the heart rate to increase (between 10 – 20 seconds). When the heart rate increases, the blood pressure approaches resting levels. Later (between 25 and 35 seconds), when blood pressure returns to normal, the heart rate declines. The 30:15 ratio, (R-R interval at beat 30) / (R-R interval at beat 15), has been recommended as an index of cardiovagal function.50, 57
The three tests mentioned above assess cardiovagal function. They are secure, useful, and economical and have great sensitivity and specificity. The tests have good standardization.
Figure 1
Simplified baroreflex anatomical and functional diagram demonstrating the baroreflex reactions to a rise in blood pressure (hypertension). BP, blood pressure; IX, glossopharyngeal nerve; X, vagus nerve; NTS, nucleus tractus solitarii; NA, nucleus ambiguous; CVLM, caudal ventrolateral medulla; RVLM, rostral ventrolateral medulla DMNV, a dorsal motor nucleus of the vagus; and IML, intermediolateral column of the spinal cord
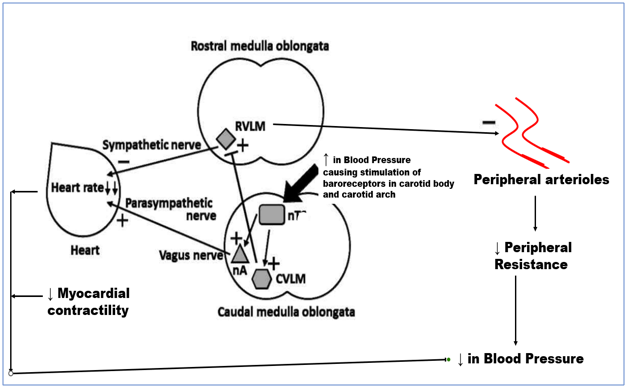
Table 1
Actions of two limbs of the peripheral autonomic nervous system.
Laboratory Indices of adrenergic Function
Valsalva maneuver's BP recordings
Recording during phases of the Valsalva Maneuver in the clinical laboratory has been made possible by the availability of a well-validated photoplethysmographic volume clamp method to quantify beat-to-beat BP. 36, 58 The test significantly improves the laboratory assessment of adrenergic function's sensitivity and specificity.57
Isometric handgrip test (IGT)
Both systolic and diastolic blood pressure and heart rate increase with prolonged muscular contraction during IGT. The central nervous system and muscular contractions provide the stimulation. 59 Efferent fibers reach the muscle and heart, increasing cardiac output, blood pressure, and heart rate.
Lying to a standing test
Hemodynamic studies have shown that there is an initial drop in BP on active standing caused by systemic vasodilatation, which in turn is caused by activation of cardiopulmonary baroreflexes as a reflex response to the rapid return of blood to the heart resulting from contraction of the abdominal and lower extremity muscles that compress splanchnic and muscular vessels. 60
Autonomic Challenges and Methods of Evaluation
Disturbances of the autonomic nerve system influence a significant part of the pathophysiology and clinical development of many diseases. In recent years, the clinical evaluation of autonomic function has advanced quickly. The monitoring of autonomic dysfunction has been described as using a variety of approaches as diagnostic tools.
Mental arithmetic
This test uses serial subtraction (often 100 minus 7 or 1000 minus 13) to activate sympathetic outflow. Systolic blood pressure should rise after that by more than 10 mmHg. 61
The cold pressor test
Due to the activation of afferent pain and temperature fibers from the skin and emotional arousal, immersion of hands or feet for 60 to 90 seconds in cold water (4°C) should result in sympathetic activation and increased blood pressure and heart rate.62
Paced breathing
Breathing is a vital process that functions automatically but can also be voluntarily controlled to reach specific goals, especially preventive and therapeutic ones.63 Heart rate variability (HRV) is widely used in studies to investigate non-invasively the effects of paced breathing at 0.1 Hz on cardiac vagal activity and the activity of the vagus nerve regulating cardiac functioning.63, 64, 65
Most HRV parameters are affected by the contribution of the sympathetic (SNS) and parasympathetic nervous systems (PNS). However, some specific HRV parameters are exclusively affected by the PNS contribution to cardiac functioning (referred to as cardiac vagal activity).58, 66, 67 It is well established that RMSSD measures PNS activity in the time domain and the frequency domain by either LFabs or HFabs, depending on the frequency of breathing. Particularly, cardiac vagal activity is seen in HFabs when the breathing frequency is between 9 and 24 CPM 58, 67 and LFabs when the breathing frequency is lower than 9 CPM (86). Paced breathing at 0.1 Hz leads to an increase in the parasympathetic nervous control of the heart and is associated with an increase in RMSSD and LFabs. 67, 68, 69
Valsalva Maneuver
The Valsalva Maneuver comprises an abrupt transient voluntary elevation of intrathoracic and intra-abdominal pressures provoked by straining. The Valsalva ratio is calculated to evaluate the cardiovagal heart rate responses. During phase 2 of VM, there is tachycardia; during phase 4, there is rebound bradycardia. Latency for the highest tachycardia is the time elapsed from the beginning of the Maneuver till the appearance of the highest tachycardia in phase 2, and latency for bradycardia is measured as the time elapsed from the end of the Maneuver till the appearance of the highest bradycardia during phase 4. These are called peak tachycardia and peak bradycardia latencies, respectively.70
Graded head-up tilt testing
Many researchers have recorded the blood pressure and heart rate responses to graded head-up tilt, i.e., sequential tilting at various degrees from 0° to 90°. Lim et al., 2013 reported a nonlinear increase in Heart rate with progressive tilting (27.5% increase at 80° HUT) with a steeper/greater increase at higher tilt angles. 71 On the contrary, cardiac output showed an opposite trend, where it decreased nonlinearly with increasing tilt angles, with a greater reduction at low tilt angles. Cardiopulmonary baroreceptors, sensitive to a slight fall in preload or central venous pressure, contribute to the nonlinearity observed in the cardiac output response to progressive tilting. This explains the experimental findings by Tuckman et al., who found that the decrease in cardiac output in normal men tends to reach its maximum at 20° with a smaller decrease between 20° and 90°. 72 Similar findings were reported by Critchley et al., 1997, that heart rate decreased with small angles of tilt (10°) but increased with larger angles of tilt (30 and 55°). They also reported that MAP and Systolic Arterial Pressure starts increasing only after tilting to 20°. 73
Yokoi et al., 1999 reported that the sympathovagal balance, as determined by (the low-frequency/high-frequency LF/HF) ratio increased with a significant difference between 0° v/s 90° and 30° v/s 90°. On the contrary, their study reported no significant changes in SBP (systolic blood pressure) and MBP (mean blood pressure). 74
Junichi et al. reported a significant increase in the LFnu and the LF-HF ratio during graded tilting and concluded that 60° HUT causes sympathetic activation, parasympathetic withdrawal, and sympathetic dominance in sympathovagal balance. 75
Bouhaddi et al. performed graded HUT in twenty healthy subjects. They concluded that DBP consistently reflects vascular sympathetic activation correlated with plasma catecholamine levels and MSNA activity. However, such a graded incremental profile was not observed in SBP. 76
Head-down tilt test
The head-down tilting (HDT) bed rest involves having the individual lie on a tilted head-down (often 6° from the horizontal), a common analog used to simulate the physiological consequences of spaceflight on Earth. So far, 6° HDT has been used as a model for weightlessness, which causes fluid redistribution in the human body. It makes it easier to eliminate gravity's effects on the human body, similar to those caused by astronauts' exposure to microgravity. According to research done by Fu et al. (2000) on the effects of 6° HDT on the cardiovascular system, the mean arterial pressure did not change, the heart rate (HR) was reduced by 9%, the stroke volume (SV), and the cardiac output (CO) increased significantly.77
According to Shankhwar et al., a tilt level of 6° head-down is ideal for creating microgravity on Earth and measuring instantaneous cardiovascular parameters. Increased vagal activity and CO are present, but cardiac sympathetic activity is not activated.
It was observed that the high-frequency power in HRV was increased at 6° HDT with a concomitant reduction in the low-frequency power of HRV. 78
Clinical Significance of Autonomic Insufficiency
Any illness that affects the central or peripheral autonomic nerve system might cause autonomic insufficiency. As with the syndrome of primary autonomic dysfunction, the disorders may entail the primary (idiopathic) degeneration of autonomic postganglionic fibers without additional neurologic abnormalities. 79 Autonomic dysfunction is more frequently a side effect of another known illness. Diabetes mellitus is the disease entity that is most frequently connected with, and it is typically accompanied by blatant peripheral polyneuropathy. Porphyria, vitamin B12 insufficiency, Wernicke's encephalopathy, and alcoholism are other dietary or toxic/metabolic conditions that can cause autonomic dysfunction. Orthostatic hypotension brought on by autonomic dysfunction is a common hallmark of amyloidosis neuropathy and can also be present in tabes dorsalis, generally in conjunction with other typical tabetic symptoms. 80 Central causes, such as posterior fossa tumors, cerebral vascular accidents, central hemorrhages, and syringomyelia, can also disrupt the autonomic reflex arc.
Care must be taken to distinguish between autonomic dysfunction and poor postural adjustment of blood pressure in older people. 80, 81 The intensity of orthostatic symptoms, their responsiveness to treatment, and, most importantly, the prognosis of the underlying disease process all influence the relevance of autonomic dysfunction due to other disorders. The prognosis for the primary types of autonomic dysfunction, peripheral and central, is typically poor, particularly for those accompanied by movement abnormalities or Parkinsonian symptoms. The average age at which these primary syndromes manifest is in the sixth decade, and less than 50% of patients survive five years after the onset of neurologic symptoms.79, 80, 81
Conclusion
The autonomic nervous system (ANS) may influence CA abnormalities, among other physiological mechanisms. The role of the ANS in controlling CBF is hotly contested due to several factors, including the complexity of the ANS and cerebrovascular interactions, measurement flaws, differences in methods used to evaluate the ANS regarding CBF and experimental strategies that can or cannot shed light on the sympathetic control of CBF. It may be beneficial to investigate the role of the autonomic nervous system (ANS) on CA to comprehend better the mechanisms underlying the detected interaction between cardiovascular and cerebrovascular regulations, define more precisely the frequency bands to compute spectral CA markers, and develop models more in line with physiology to describe the behavior of CA. The review examines how the ANS impacts CA, which could significantly impact how pathological disorders are diagnosed and treated. Autonomic insufficiency may result from any disease that affects the central or peripheral autonomic nervous system. The illnesses may involve the main (idiopathic) degeneration of autonomic postganglionic fibers without other neurologic abnormalities, similar to the syndrome of basic autonomic dysfunction.